The Additive Manufacturing (AM) of aluminium alloys is facing a growing development mainly thanks to the optimization of the production process and of the quality of the powders used as raw material. this topic arouses lively interest in both the scientific community and in the industrial world. even if AM features undisputed advantages compared to conventional technologies, certainly it cannot represent a competitive alternative for several applications. nevertheless, it is very interesting to ASSESS what performances are actually provided by the components manufactured through AM, in comparison, for instance, with items obtained through foundry techniques.
At Brescia University, they are carrying out a study on the characterization of the components manufactured with two different processes, AM and gravity casting, with the same AlSi10Mg alloy. The use of powders of this material for the additive manufacturing is in fact particularly diffused, as well as the use of this alloy in foundry. This study is framed in a broader context of researches in progress to study in-depth properties and possible applications of metal components, like for instance titanium and aluminium alloys, produced through additive manufacturing in comparison with conventional processes. Characterization activities are concerning a wide variety of properties of those materials under different heat treatment conditions, to make a constant parallel comparison to identify better potentialities and criticalities.

In Figure 1, we report first of all the typical microstructure of AlSi10Mg alloy samples obtained respectively through gravity casting and AM before the heat treatment (conditions as-produced). Concerning the additive technology, they have used a machine that exploits the process called Selective Laser Melting (SLM). We can immediately notice how the two considered processes actually lead to extremely different microstructures. In Figure 1a, we recognize a dendritic microstructure, where the aluminium matrix is surrounded by the eutectic phase, and we can identify also some intermetallic compounds. In Figure 1b, it is instead possible to identify the so-called “liquid puddles” associated to the melting and solidification of successive portions of material due to the progressive laser action. The edges of these zones are generally visible only after opportune etching with chemical reagents. Inside these areas, it is not possible to distinguish different phases, at least with the considered magnification. Deeper analyses have led to the identification of an extremely fine network of Si particles inside the aluminium matrix. After the initial characterization phase of the two materials, they have decided to proceed to the execution of heat treatments of solubilisation, hardening and ageing (T6 heat treatment). This represents a standard procedure for foundry components manufactured with alloys of the Al-Si-Mg family, with the target of strengthening the material and improving its mechanical characteristics. Concerning this, the debate on the efficacy of conventional heat treatments for the components produced through AM is still open. In fact, due to the peculiar microstructure, they are trying to define some specific heat treatments for this type of material, to exploit its potentialities at best. It is very often suggested, for instance, to apply a stress relieving treatment instead of a T6 treatment. However, standard parameters of temperature and treatment times have not been defined, yet. Concerning the analyses carried out by Brescia University, they have opted for the execution of the same heat treatments for both manufacturing processes considered, in order to compare directly materials’ performances. About this matter, if we compare the microstructural characteristics of the two material typologies under the same heat treatment conditions, we can notice evident differences.
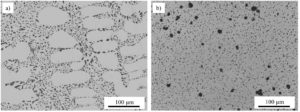
As shown in Figure 2, for the material produced through additive manufacturing, the treatment at high temperature mainly causes:
- Loss of the fine microstructure and of “liquid puddles”,
- Coarsening of Si particles,
- Growth of the sizes of gas porosities.
More in detail, we must consider that the heat treatment analysed reduces the dependence of the mechanical material properties on the growth direction given by the peculiarities of this manufacturing process, making such properties more uniform. On the other hand, the extremely fine microstructure that is obtained because of high cooling speeds downstream the laser action is not maintained after solubilisation, thus causing a resistance decrease of the material itself. During the solubilisation, the diffusion of Si atoms occurs, too. This causes the fragmentation and the spheroidizing of the particles of eutectic Si in foundry alloys, whereas for AM we can notice a coarsening of these particles, which reach a diameter of some micrometres, whereas in conditions as-built they have smaller sizes and they are interconnected one another in a sort of network. The successive ageing causes anyway the formation of hardening precipitates (phase Mg2Si), likewise what happens for the alloy manufactured through conventional technologies. In terms of hardness, the two materials follow two opposite trends. As well known, after the T6 heat treatment, the components produced through gravity casting increase their hardness whereas, regarding the AM material, after the heat treatment, the value measured under as-built conditions is not exceeded for any of the combinations of temperature and time applied. As a matter of fact, in the studies carried out they have tested numerous combinations of temperature and time, concerning both solubilisation and ageing. Solubilisation substantially reduces the hardness of the AM material and the successive ageing is not sufficient to exceed the values under conditions as-produced, whereas the material performance complies with the one of the samples obtained through gravity casting.
After a first optimization of such parameters according to macro-hardness measurements, they have afterwards carried out some traction tests under some of the heat treatment conditions chosen. As anticipated, one of the effects connected with the heat treatment of solubilisation for AM is the size increase of the porosities formed in the material during the process. Very likely, they contain gas coming from the process itself that, after the exposure to high temperatures, deforms the material determining a coarsening of such porosities. The sharing of these defects in the material performances is certainly an important parameter worth considering. Nevertheless, we can notice how the tensile strength is anyway significant also after the T6 heat treatment. The efficacy of this treatment for the material manufactured in conventional manner is such as to have comparable performances with the material obtained through additive technology, with the same heat treatment. The microstructural aspect has been taken strongly into account also concerning the characterization of the impact behaviour of the two materials through Charpy resilience test. The study, carried out in collaboration with Ferrara University, has allowed analysing thoroughly the fracture mechanism when the manufacturing technology and heat treatment parameters vary. In addition, they were also committed to study the effect of the HIP (Hot Isostatic Pressing) treatment applied according to different modalities, with the target of decreasing the porosity coarsening during the heat treatment. The HIP process provides for the material exposure to high temperature and to high isostatic pressure, in order to act uniformly on all directions and to contrast the rise of gas porosity sizes. Concerning the resilience of the materials manufactured with different technology, they have observed a much better behaviour of AM samples in comparison with cast ones. AM samples proved to be able to absorb a higher quantity of energy associated to the impact versus the samples produced through gravity casting in all considered conditions. As expected, the HIP treatment has led to a further increment of these parameters. Finally, they have also evaluated the cavitation resistance of such materials.
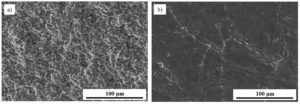
Cavitation phenomena can in fact arouse in some particular applications and they can represent a highly critical situation for some components. In this case, they have observed that the fine microstructure of AM samples before the heat treatment is apt for assuring a very high resistance to such phenomenon and a neatly inferior erosion speed also to the same material after the heat treatment. These results can be particularly interesting in view of widening the possible applications of AM components. On the other hand, after the T6 treatment, we can notice that material performances become uniform, irrespective of the manufacturing process used. The HIP process allows instead improving the resistance of AM samples after heat treatment, even if the material performances under conditions as-produced are not equalled.
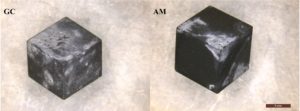
Finally, the characterization has been completed considering also the resistance to corrosion in saline environment. Immersion tests have highlighted a highly critical aspect for AM in comparison with cast items. In fact, in this case the particular microstructure implies a corrosion mechanism that, once triggered, leads to high weight losses in comparison with the same alloy manufactured with foundry techniques. This criticality is strongly decreased after the T6 heat treatment, which in this case represents a fundamental treatment to improve the resistance in view of applications in aggressive environments. These are some examples of analyses still in progress to study in-depth the effective properties of the materials produced through AM technology in direct comparison with the counterpart obtained through conventional processes. In this way, it is possible to provide a more critical vision of the influence of the manufacturing process on the material performances, to identify specific potentialities and possible innovative applications.